En respuesta al anuncio del alcalde Ismael Burgueño Ruiz sobre...
Leer más
Treatment of Genetic Diseases With CRISPR Genome Editing
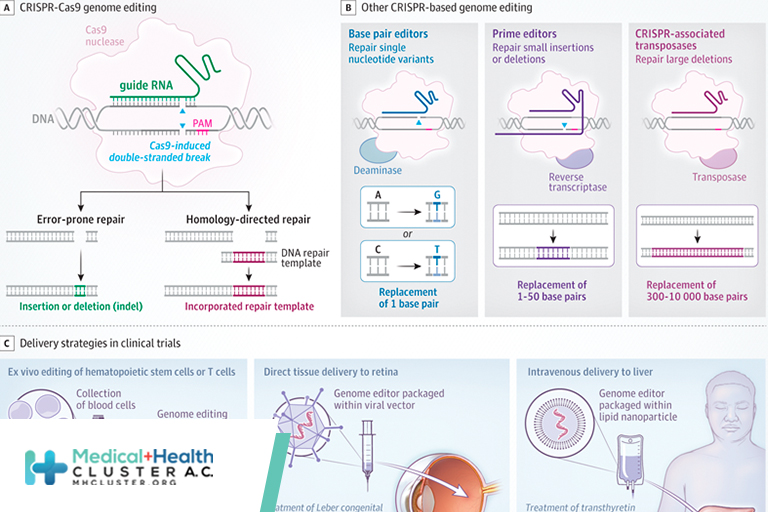
In nature, microorganisms use CRISPR (clustered regularly interspaced palindromic repeats) and CRISPR-associated (Cas) proteins for antiviral immunity through recognition and destruction of specific DNA sequences. Over the past decade, CRISPR genome editing has been developed to create transformative technologies to treat, cure, and prevent human disease.
How CRISPR Works
CRISPR genome editing allows scientists to change DNA sequences in cells at virtually any desired position, enabling both fundamental research and therapeutic applications (Figure). CRISPR-Cas9, the most widely used genome editor, is an RNA-guided DNA-cutting enzyme that makes double-stranded DNA breaks at preselected (on-target) positions in the DNA of living cells.1 Repair of the break site results in either small insertions and deletions (indels) introduced by error-prone repair or insertion of a new DNA donor sequence chosen by the investigator (homology-directed repair). Indels are useful for interrupting gene function, whereas sequence insertion can replace a defective sequence to restore gene function.2 In either case, controlling the exact editing outcome for a particular indication in a specific cell type or organ is challenging, and unintended (off-target) DNA changes can be harmful.
New Genome-Editing Strategies
Several proteins related to Cas9 have been discovered or engineered to better control editing outcomes. Although most of these tools have not yet been advanced to clinical use, they offer a window into the future of precision genome editing. These include genome editors that can change single nucleotides (base editors) to repair single nucleotide variants,3 replace short (<50 nucleotides) stretches of DNA (prime editors) to fix small insertions or deletions,4 or insert large DNA sequences over 1000 nucleotides long (Cas transposases) to address large deletions.5 Other tools allow for control of gene expression without permanent changes to DNA, including fusion of Cas9 lacking DNA cleavage activity to transcriptional regulators that transiently inhibit (CRISPRi) or activate (CRISPRa) gene transcription. Similar fusion enzymes change epigenetic markers, such as histone acetylation/methylation, allowing for long-lasting effects on gene expression (CRISPRon, CRISPRoff).5 Additional engineered versions of Cas9 with different properties that affect both activity and cellular delivery may soon be suitable for clinical use.
CRISPR Editing in Clinical Trials
Ongoing clinical trials use CRISPR for somatic cell genome editing to treat hereditary diseases or cancer. In these studies, cells are either removed and edited in tissue culture and then readministered to the patient (ex vivo), or genome editors are packaged within viral vectors or lipid nanoparticles and given intravenously to home to specific tissues (in vivo). Ex vivo therapies have focused on blood disorders, including at least 6 trials aiming to cure sickle cell disease (SCD) or transfusion-dependent β-thalassemia (TDT), both of which are caused by variations in the hemoglobin beta (HBB) gene (NCT03655678; NCT03745287; NCT04443907; NCT04774536; NCT04819841; NCT04853576). This can be achieved through increasing fetal hemoglobin expression relative to the mutant beta globin, or by direct correction of the causative HBB variants. In a pivotal trial, 11 patients with SCD have been followed up 1 to 3 years after autologous hematopoietic cell transplant with durable editing that substantially decreased the frequency of vaso-occlusive crises with no significant adverse events (SAEs).6 Clinical trials have also started for cancer immunotherapy using ex vivo edited T cells7,8 (NCT03399448, NCT04637763, NCT04035434, NCT04426669, NCT04244656, NCT02793856) and type 1 diabetes using engineered pancreatic cells (NCT05210530).
Beyond ex vivo genome-editing applications, there is now strong proof of concept for in vivo use. Five patients have been treated for Leber congenital amaurosis, a disorder of the retina, a subset of which is caused by an aberrant intronic splice site in CEP290. Viral vector delivery of genome editors by retinal injection allows for removal of the inappropriate splice site. Thus far, patients have had mild clinical improvement without dose-limiting toxicity or SAEs (NCT03872479). Two trials are using intravenous administration of lipid nanoparticles that contain CRISPR/Cas9 mRNA and preferentially home to the liver to reduce liver protein production. The first indication is for transthyretin amyloidosis (ATTR), in which mutant protein causes toxic amyloid aggregates (NCT04601051). So far, 15 patients have received CRISPR-Cas9 lipid nanoparticles to generate indels in the TTR gene, leading to at least 6 months of durable editing, decreased serum TTR, and no toxic effects or SAEs.9 A similar strategy is being used to reduce kallikrein B1 (KLKB1) for hereditary angioedema (NCT05120830).
Challenges and Ethical Considerations
The advancement of in vivo genome-editing therapies will require genome editors that are not only precise, but limit off-target edits at unintended genomic loci. New assays need to be developed to monitor efficacy and safety, particularly against unintended edits that may cause tumorigenesis or chromosomal rearrangements. New methods of in vivo delivery will be required to improve pharmacologic distribution and achieve tissue specificity, particularly to address neurological and systemic diseases. Diagnostics to measure on- and off-target tissue delivery need to be developed. Regulatory agencies will need new approval pathways to address therapeutic candidates for more than 180 000 known pathogenic gene variants, many of which are rare and not amenable to traditional clinical trials, and as genome-editing technology advances the FDA-recommended long-term follow-up of 15 years may become impractical or unnecessary. Future applications of genome editing will expand beyond rare inherited diseases to common indications, such as reduction of hepatic PCSK9 protein levels to lower cholesterol.10 As capabilities in genome editing progress, ethical challenges include editing of alleles that do not directly cause disease, editing of germline cells for inherited diseases, and equitable access to therapy. Scientists and clinicians should engage in ongoing discussions about responsible use and regulation of genome editing to safeguard the tremendous potential of CRISPR technologies to treat, cure, and prevent disease.
Conflict of Interest Disclosures: Dr Kan reported being a fellow in the Pediatric Scientist Development Program, supported by award K12-HD000850 from the Eunice Kennedy Shriver National Institute of Child Health and Human Development. Dr Doudna reported being a cofounder of Caribou Biosciences, Editas Medicine, Scribe Therapeutics, Intellia Therapeutics, and Mammoth Biosciences; serving as a scientific advisory board member for Caribou Biosciences, Intellia Therapeutics, eFFECTOR Therapeutics, Scribe Therapeutics, Mammoth Biosciences, Synthego, Algen Biotechnologies.
Créditos: Comité científico Covid